My Projects
Maximum entropy theory in ecology
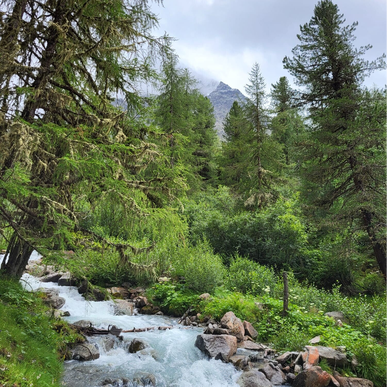
Maximum entropy theory of ecology (METE) models can predict state variables and as such infer processes occurring in ecological communities. For example, they can predict the shape of species-abundance distributions, species-area relationships, and occupancy-abundance relationships extremely well while considering only limited input. It has been shown that, while stable ecosystems can be properly described using maximum entropy theory, ecosystems undergoing changes (e.g. unstable ecosystems) deviate from the predicted relationships.
In this project, I investigate whether deviations from maximum entropy predictions relate to the type and magnitude of disturbances occurring in the ecosystem. Linking observed deviations to disturbance type and magnitude in study systems may provide us with more knowledge on the state of any other ecosystem for which data on ecological community composition is available. By inferring an ecosystems state, we may be able to propose conservation and restoration measures that are required to stimulate ecosystem recovery towards a healthier state.
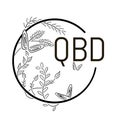
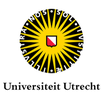
Optimizing time intervals for camera trapping analysis
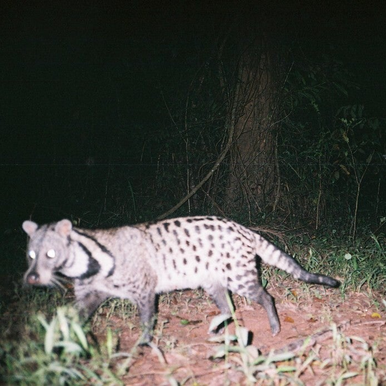
To direct conservation efforts, species abundances need to be estimated accurately. Camera trapping data are often used for this purpose, but obtaining adequate approximations of species abundances is difficult for species in which individuals cannot be identified. By using the Royle-Nichols model (Royle and Nichols, 2003), a species’ occupancy or local abundance can be compared between sites, without the need for mark-recapture data.
Occupancy models require that continuous detection histories are condensed to time intervals with either detection or non-detection. Most researchers arbitrarily set this time interval at 5 days. However, the chosen time interval will have a substantial effect on the model’s outcomes. What time interval is adequate depends on the species’ actual abundance and the movement behavior of the individuals. Currently, there is no model that takes into account a range of time intervals to estimate local abundances.
In this project, we aim to optimize the choice of time interval and adjust the Royle-Nichols model to automatically incorporate a range of time intervals. The new model will provide more accurate estimations of local abundances per species and less bias in what time interval the user thinks should be appropriate.
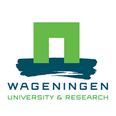
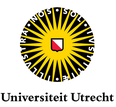
modelling goose migration
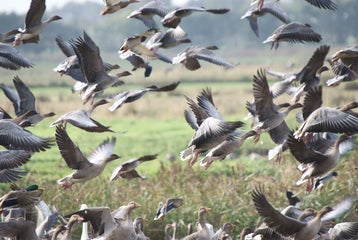
Species have to adapt to anthropogenic changes, such as global warming, hunting, and land use changes. While population sizes of many species are rapidly decreasing as a consequence of these human-induced changes, others are increasing. For instance, the population size of pink-footed geese has shown a substantial rise over the last 40 years. With larger population sizes, a number of issues arises. Both demographic changes and environmental changes may induce rapid evolution of behavioral traits. However, how these different changes interact and affect goose behaviour is unknown. I will model these processes in a spatially explicit way, in order to gain more insight in eco-evolutionary dynamics of the Pink-Footed Goose.
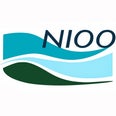
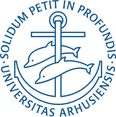
Modelling Maui dolphin movement
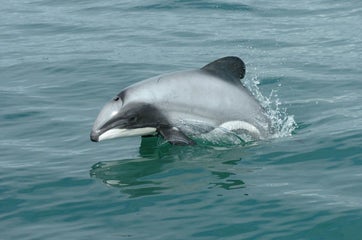
Anthropogenic impacts on nature are countless, enormous, and often absolute. Yet, some deleterious effects can still be overcome when man-made stressors are removed from the environment. In order to implement successful restoration measures, accurate predictions are needed. In this project, I collaborate with Prof. Liz Slooten (University of Otago, New Zealand), Geerten Hengeveld (Biometris, Wageningen University and Research), and Wolf Mooij (NIOO) to generate and calibrate a tailor-made agent-based model, which will be used to predict how well regulations of commercial fishery activities will reduce the impact of fishing on a highly endangered dolphin species, the Maui dolphin.
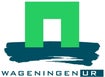
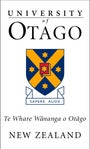
Evolution of hydrochorous seed dispersal
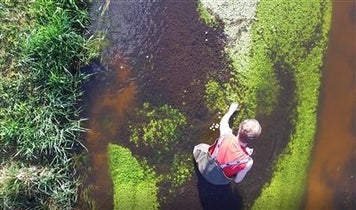
At Utrecht University, I collaborate with Prof. Merel Soons on seed dispersal by water in lowland streams. During this project, I aim to answer the following questions: 'How can plants optimise seed dispersal via water?' and 'What are the effects of stream characteristics, such as meandering or vegetation, on seed dispersal?'. See our publication in Functional Ecology here.
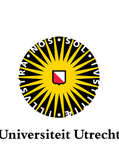
Evolution of seed dispersal strategies
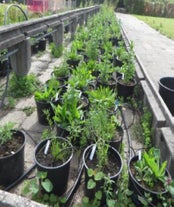
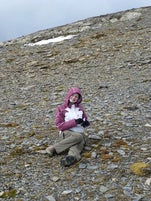
Plants can, by altering their (seed) characteristics, affect their seed dispersal kernel. As plants with seed dispersal kernels better suited to the environment get more seeds to suitable habitat, their frequencies increase over time. Such evolution of seed dispersal strategies can vary widely between species and between environments. See our papers in Oikos here and Journal of Ecology here.
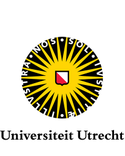
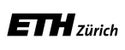
Cooperation in mussel beds
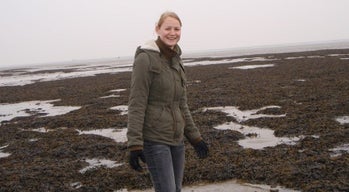
Mussels, like many organisms, cooperate with each other to decrease predation and other risks. During my PhD at the Royal Netherlands Institute for Sea Research (NIOZ), I examined the pros and cons of cooperating and cheating in self-organized mussel beds. See our publication in Evolutionary Ecology here.
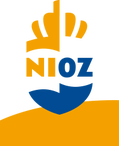
eco-evolutionary dynamics of mussel movement strategies
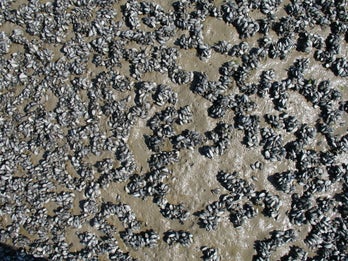
Mussels self-organize into labyrinth-like patterns to decrease predation risk (by aggregating into dense clusters) while maintaining the flow of algae-rich water through the mussel bed (hence the gaps). Moving into a labyrinth-like pattern can be done most efficiently when using a Levy walk-like movement strategy (see our Science publications here and here). Observed movement patterns become more Brownian-like with increasing mussel density, as encounters truncate the steps (see our Proceedings of the Royal Society B papers here and here).
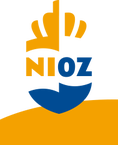